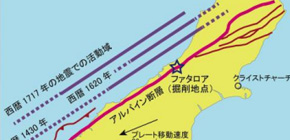
Sampling of the Active Alpine Fault in New Zealand Reveals Extreme Hydrothermal Conditions
International research team involving Naoki Kato of Osaka University identifies anomalous temperature and pore fluid pressure gradients at shallow depth, which affect mineral and seismic stability in the active Alpine Fault of New Zealand
A recent study published in Nature has demonstrated unusual heat generation and fluid movement in the Alpine Fault of New Zealand that has implications for understanding earthquakes in the region. Large plate-boundary faults, such as the Alpine Fault, are important areas of stress build-up and release, which can lead to earthquakes. There is increasing evidence that faults in such regions have lower than predicted frictional shear strength, and are subject to very limited heat generation during fault slippage.
To investigate fault strength and heat generation in the Alpine Fault, the Deep Fault Drilling Project (DFDP) was commissioned. “The primary motivation of the DFDP was to provide an understanding of the ambient conditions, rock properties and geophysical phenomena that occur immediately before a large earthquake, because we just don’t know enough about active faults before they rupture” says Naoki Kato of the Department of Earth and Space Science, Osaka University who co-authored the work.
The research team drilled to a depth of 893 m directly into the active Alpine Fault in Whataroa, New Zealand. The fault moves at about 26 mm per year and has, over time, brought rocks to the near-surface from depths of 30 km. Various geophysical techniques, including fiber optics, were used to obtain very precise temperature measurements, and revealed a pressure gradient almost 10% greater than expected, and temperature gradients (>80 °C.km -1 ) more typical of active volcanic regions.
The temperature structure of the fault was modeled in terms of heat conduction, rock advection and fluid advection related to topography. “Our models show that rock advection and thermal diffusion are the primary heat transport mechanisms in the principal slip zone, and it is fault slip itself that brings both rock and heat up from depth” Naoki Kato says. The models and drilling data both show that lateral fluid movement transports significant amounts of heat and fluids from depth, both of which concentrate into valleys.
Heat generation and fluid migration is important in active faults because both directly affect the stability of phyllosilicate minerals (clays), thermal rock expansion and the formation of physical and chemical reaction products in the fault slip zone. These in turn control the frictional and mechanical behavior of faults, and therefore the behavior of earthquakes that may occur during slippage. This study sheds new light on earthquake development in active fault regions because shallow temperature and hydrothermal anomalies, and their lateral variation, affect dynamic strength along the length of the fault.
Abstract
Temperature and fluid pressure conditions control rock deformation and mineralization on geological faults, and hence the distribution of earthquakes 1 . Typical intraplate continental crust has hydrostatic fluid pressure and a near-surface thermal gradient of 31 ± 15 degrees Celsius per kilometre. At temperatures above 300–450 degrees Celsius, usually found at depths greater than 10–15 kilometres, the intra-crystalline plasticity of quartz and feldspar relieves stress by aseismic creep and earthquakes are infrequent. Hydrothermal conditions control the stability of mineral phases and hence frictional–mechanical processes associated with earthquake rupture cycles, but there are few temperature and fluid pressure data from active plate-bounding faults. Here we report results from a borehole drilled into the upper part of the Alpine Fault, which is late in its cycle of stress accumulation and expected to rupture in a magnitude 8 earthquake in the coming decades. The borehole (depth 893 metres) revealed a pore fluid pressure gradient exceeding 9 ± 1 per cent above hydrostatic levels and an average geothermal gradient of 125 ± 55 degrees Celsius per kilometre within the hanging wall of the fault. These extreme hydrothermal conditions result from rapid fault movement, which transports rock and heat from depth, and topographically driven fluid movement that concentrates heat into valleys. Shear heating may occur within the fault but is not required to explain our observations. Our data and models show that highly anomalous fluid pressure and temperature gradients in the upper part of the seismogenic zone can be created by positive feedbacks between processes of fault slip, rock fracturing and alteration, and landscape development at plate-bounding faults.
Figure 1. Distribution of the Alpine Fault.Star symble, drilling site. (credit: Osaka University)
To learn more about this research, please view the full research report entitled " Extreme hydrothermal conditions at an active plate-bounding fault " at this page of the Nature website.
Related links