The Reality of Quantum Computer Use: Computational Chemist Pursues Quantum-Classical Hybrid Algorithm
Specially Appointed Associate Professor (Full-time) Wataru Mizukami Institute for Open and Transdisciplinary Research Initiatives
The term “quantum computer” used in the context of “When will a quantum computer be realized?” often refers to an “all-purpose (almighty)” quantum computer with an ultra-high calculational capability that possesses the function of self-correcting errors in calculation results, which are also seen with the existing computers we routinely use at present. Now, the competition over research and development of quantum computers is intensifying worldwide. However, an additional two or three decades are estimated before a complete form of a quantum computer becomes available. If you now feel “I see. That is a dream story,” you are the very person for whom I recommend reading this article until the end. In around 5 years from now, it will be possible to utilize the amazing calculational capability expected of quantum computers in “some fields” before a complete form becomes available. Application of such a capability to the field of quantum chemistry is particularly attractive because of its large impact on the market and the availability of the research results accumulated over the past 90 years. “Chemistry”, which pertains to pharmaceutical and other industrial products, metabolism inside the living body, etc. is closely related to our daily life. There is a large demand for calculations for the prediction of complex chemical reactions and many mechanisms of chemical reactions remain unclarified even with the supercomputers currently available. Then, how can we utilize the power of an uncompleted quantum computer? This question will be answered by Specially Appointed Associate Professor (Full-time) Wataru Mizukami, Center for Quantum Information and Quantum Biology, Osaka University (QIQB), who is actively engaged in the development of new algorithms for computational chemistry (hereafter referred to as “Associate Prof. Mizukami”). The birth of an “ultimate microscope” enabling visualization of macroscopically invisible chemical reactions with a quantum computer is near.
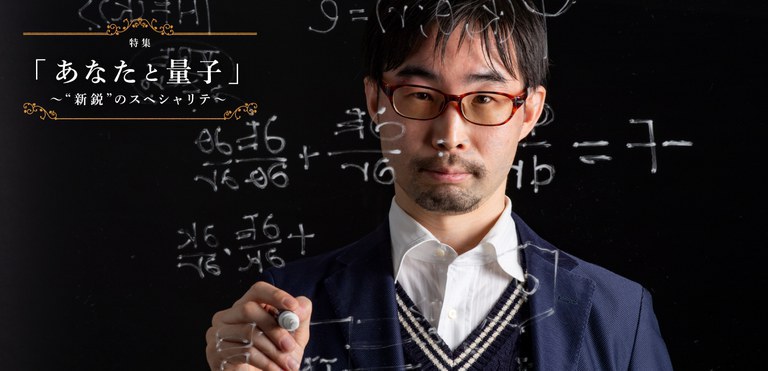
Make invisible chemical reactions visible through calculation
It is said that approximately 40% of all calculations currently performed with supercomputers pertain to the chemical field. This is because complicated calculations need to be performed at a high speed in studies involving investigation of the structure of materials, the way of interactions among molecules during chemical reactions and exploration of candidate substances for drugs.
Associate Prof. Mizukami says: “Difficulties we face in chemistry include the size of molecules being too small and motion of molecules being too fast. For example, the diameter of a hydrogen atom is 1.1 ten-billionth of a meter (1.1×10-10 m). This means that when 1 m is expanded to the diameter of earth, the hydrogen atom is only about the diameter of spaghetti (1.4 mm). This resembles viewing the spaghetti on the ground from space. It is quite small. Furthermore, when hydrogen and oxygen vibrate, their speed is one 100-trillionth of a second (10×10-15 sec). If this is counted as 1 second, one second in our world corresponds to 3.2 million years. Molecules are thus small and fast-moving. It is highly difficult to observe such small objects moving at such a high speed. However, if simulation by calculation is possible, we can reproduce the chemical reactions at a scale macroscopically visible for us. Then, we may have “an ultimate microscope” that converts the invisible world into one visible in detail.”
Course of the 90-year period was beyond Dirac’s imagination
The basic rules underlying the calculation of atomic and molecular behaviors were established by completion of quantum mechanisms at the beginning of the 20th century. Paul Dirac (1902-1984, Nobel Prize in Physics laureate in 1933), who is one of the founders of quantum mechanics, stated in 1929 that all basic rules for mathematical processing of chemistry are known. The first principle on quantum chemical calculation is one such rule. However, knowing the equation for describing a given chemical phenomenon does not always allow us to find a solution to that equation. Associate Prof. Mizukami commented on this, saying: “Dirac made such a statement probably meaning: ‘A solution to the equation may be found if many efforts are made, but the equation is practically too complex to solve.’”
Chemistry handles a limitless number of molecular combinations. As the number of molecules constituting a material increases, the calculation becomes exponentially more complex. For this reason, chemists have been continuing to make efforts, including replacement of the complex reactions of materials with a simpler model, creating an approximate equation easier to calculate instead of finding a strict solution to a given equation. Thanks to such wisdom and ideas of researchers after the era of Dirac, the precision of the quantum chemical calculation has been improved.
Truth revealed by simulation
Owing to advances in algorithms (a process or set of rules for calculations) and improvements in the calculation capacity of computers themselves, it became possible to apply computer simulation as a means of solving chemical problems in the 1970s. Pople, a theoretical chemist, developed a computer program for computational chemistry, for which he won the Nobel Prize in Chemistry in 1998.
The reaction between metallic sodium and water is an example where computational chemistry elucidated the mechanism for chemical reactions.
It is known that if metallic sodium is put into water, an explosively intense reaction will occur. However, because this reaction occurs at quite a high speed, its exact nature and mechanism remained unexplained for many years. Even the images taken with an ultra-high-speed camera failed to reveal what was happening. In recent years, however, it was revealed by computer simulation. It unveiled the hidden process composed of repeated sequences of deprivation of electrons at the moment of sodium contact with water → generation of positively charged sodium ions → intense repulsion between positively charged ions → formation of another sodium to react with water.
Through joint research with the Max Planck Institute for Polymer Research, the Osaka University Graduate School of Engineering Science, etc., Associate Prof. Mizukami himself conducted simulation using quantum chemical calculation about the behavior of the substance (TMAO: trimethylamine N-oxide) possessed by many deep sea fish that is known to make protein resistant to high water pressure. The simulation revealed the kinetics of TMAO to differ from those previously imagined.
Impression of stagnation prevailing in the field of computational chemistry
He says: “Chemical reactions are governed by the delicate difference in energy at the molecular level. An advantage of computational chemistry lies in that such a small energy difference can be argued about in this field.” However, he adds: “The precision of chemical calculation required for analysis of such a delicate energy difference is said to be 99.99%. This is slightly higher than the precision (approximately 99.8%) of current standard quantum chemical calculation and resembles a weather forecast, which may be off at a relatively high probability. It is still at a stage where we cannot fully trust in the findings and are obliged to check the calculation results with suspicion. “
One possible approach to improving the precision is to increase the cost of calculation progressively until the intended precision can be achieved. However, in cases where the electrons are markedly entangled with each other, this approach can fail because even a high-performance supercomputer cannot achieve the calculation completely. Thus, the trend for improvement in precision is stagnant at present.
To cross this hurdle, we have to wait for the introduction of a quantum computer able to calculate much information in the form of qubits (representing diverse states of micro-particles) at a time, unlike classical computers, which calculate the information expressed in bits (combinations of 0 and 1). However, qubit has shortcomings such as high susceptibility to the influence of noise and the potential of committing errors during the course of calculation. Many technical hurdles need to be cleared before a quantum computer with error-correcting function is completed.
Associate Prof. Mizukami remembers the impression he had when hearing the story about a quantum computer during his student years: “It sounded interesting but did not seem to be readily useful in chemical calculations. I thought it better to make more efforts to develop algorithms for classical computers.”
Breakthrough with a quantum-classical way hybrid approach
Variational Quantum Eigensolver (VQE), an algorithm composed of a mixture of quantum and classical elements to make up for the shortcomings of both quantum computers and classical computers, was introduced during the situation full of the sense of stagnation.
Quantum computers can perform complex calculations, but are highly susceptible to noise, resulting in a short period of normal functioning. An idea proposed to resolve this shortcoming was: (1) the results of calculation with a quantum computer are tentatively fed into a classical computer; (2) the quantum computer later utilizes the results stored in the classical computer to continue the calculation further; and (3) this sequence is repeated to manifest an effect identical to that of long-term continued operation of a quantum computer.
When the VQE theory was made public in 2014, Associate Prof. Mizukami felt that this had a high potential of cultivating a new frontier of quantum chemistry. He had this feeling under the circumstances prevailing in those years where he had begun to become aware of a limit in the potential of further progression of supercomputers facing the difficulty in further integration of electronic circuits, the consumption of huge amounts of electrical power and other problems.
“Also concerning quantum chemical calculation, no one in the 1970s or 1980s anticipated it to be proven useful later. Chemical calculation with a quantum computer is also at the startup stage now. What will happen 10 years later is known to no one. As I am a chemist, I can feel satisfied if there is progression in chemical calculation. In those days, I thought that quantum computers should be used to cross this hurdle. However, even if the computer’s power is improved, it will be useless unless it is accompanied by progression of the algorithm. I wish to create tools useful for researchers in order to contribute to advances in chemistry on the whole.” Saying so, Associate Prof. Mizukami expresses his attempt of taking the future into his hands in a realistic manner.
If a quantum computer with “quantum error-correcting” function becomes available for extensive use, what world will be realized?
“For example, it may lead to fixation of nitrogen contained in air and artificial photosynthesis. The Harbor-Bosch process for acquisition of nitrogen (a raw material of fertilizers) from air was developed early in the 20th century, leading to the increase in foodstuff production. However, it requires a high temperature and high pressure, consuming huge amounts of energy equivalent to 1-2% of the total energy consumed worldwide. On the other hand, some microbes possess a smart mechanism by which nitrogen can be yielded at room temperature (a mild condition). Modeling after this mechanism may lead to the development of an innovative catalyst.”
However, “the rose-colored future will not come soon,” says he. “Many people in the industrial sector think that quantum computers are readily available for use. However, science does not advance linearly. It will face many hurdles from now on. Challenging the difficulties and understanding the situations with mid- and long-term perspectives will be essential in facilitating progression in this field having a large potential, instead of seeing it collapse after a transient boom,” he says as a warning. “First of all, I wish to achieve something useful for chemists in 5 years, as a wind hole created in the wall, “he says so in a straightforward manner, inviting us to foresee that the dream is near coming true.
What is research for Associate Professor Mizukami?
For me, research is like travel. There is a preset goal, but the traveler does not know the path to reach it. This may be called wandering, but while I walk, I can see what was invisible for me before. My heart pounds and I feel excited. Sometimes I am fatigued after walking. Including these aspects, research is a pleasure.
● Specially Appointed Associate Professor (Full-time) Wataru Mizukami
Institute for Open and Transdisciplinary Research Initiatives/ Center for Quantum Information and Quantum Biology, Osaka University
- 2006: Graduation from Department of Industrial chemistry, School of Engineering, Kyoto University.
- 2008: Completion of First Semester of Doctoral Course at University of Tokyo Graduate School of Engineering.
- 2011: Completion of Second Semester of Doctoral Course at Department of Physics, Graduate University for Advanced Studies. Marie Curie Research Fellow at Bristol University (U.K.), Basic Science Special Researcher at Institute of Physical and Chemical Research. Teaching Assistant at Kyushu University Interdisciplinary Graduate School of Engineering Science, etc.
- Since July 2019: Current position. Ph.D. (Science). Major: Quantum chemical calculation.
(Interviewed in November 2020)