The Future is Now: Quantum Sensing Technology Applied to MRI will Change Healthcare As We Know It
Specially Appointed Associate Professor (Full-Time) Makoto Negoro Institute for Open and Transdisciplinary Research Initiatives
The global competition over space development, represented by the Apollo Program, stimulated the creation of numerous advanced technologies. Resembling such a course of technological development, the technologies cultivated through the efforts for the development of quantum computers will benefit mankind in numerous aspects. The technology for increasing the sensitivity of magnetic resonance imaging (MRI), an imaging modality now commonly used during healthcare, is one such example. Japan is said to lead the world in terms of the percentage of medical facilities equipped with an MRI apparatus designed to take images of deep areas of the human body such as the brain, blood vessels and organs. It has been playing a significant role in diagnosis at many hospitals. With MRI, electromagnetic waves are applied to the water molecules of the body and the weak signals returning from the hydrogen atoms are analyzed. Nuclear magnetic resonance (NMR) actively used for chemical analysis also functions under the same principle. With the conventional apparatus for MRI, however, the sensitivity is not high because the signals emitted from the atomic nucleus are weak. At the Center for Quantum Information and Quantum Biology, Osaka University (QIQB), Specially Appointed Associate Professor (Full-time) Makoto Negoro (hereafter called “Associate Professor Negoro”) and his colleagues succeeded in increasing the signal intensity by approximately 10,000-times at room temperature, thus cultivating a path for the development of next-generation MRI technology. Associate Professor Negoro expresses his anticipation, saying: “This will enable real-time assessment of the metabolic status, showing which parts of the body are being favorably affected by a given drug and how such efficacy is manifested. This is sure to significantly change healthcare.” The technology used for this kind of MRI has markedly advanced during the course of quantum computer development. The technology for precise control of the quantum state is called “Quantum Technology 2.0”, and it has a broad-range potential of application to quantum simulation, quantum communications and quantum sensing.
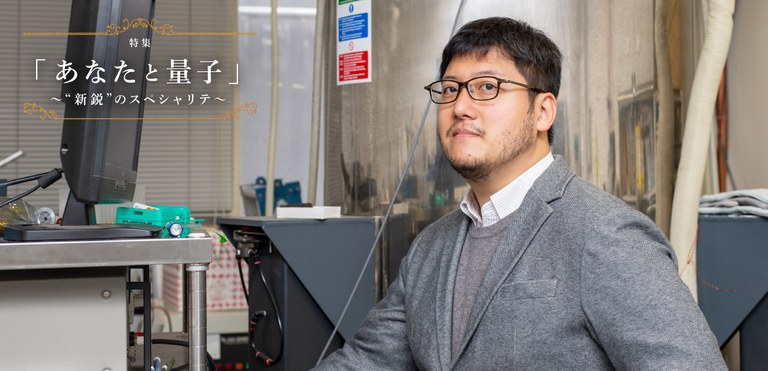
MRI sensitivity improvement by quantum technology – making up for the “weak point” of nuclear spin
Electrons and atomic nuclei have the nature of a very small magnet termed “spin.” If an electromagnetic wave is applied to a water molecule (H2O), it resonates with the nuclear spin, returning a signal. Under a normal state, however, nuclear spins align in up or down directions, and the signals from spins cancel with each other. For this reason, a strong magnetic field is applied, with the direction of magnets aligned as far as possible, but the magnetic force of the nuclear spins is weak, allowing only an approximately 1/30,000 aligning in the field direction. The polarization rate, which represents the raio of up and down directions, is 0.0033% in such a case.
Associate Professor Negoro says: “The polarization rate of 1/30,000 indicates a state where 15,000 small magnets are directed upwards and another 15,000 plus 1 magnets are directed downwards. Under such a setting, only the signal corresponding to one magnet is received for interpretation. The human body is said to contain around 40 kg of water, accounting for approximately 60% of all components. The current weak signal from MRI enables evaluation of only the motion of water abundantly present in the human body. If the signal is increased 10,000-fold, it will be possible even to evaluate the motion of a 4-g drug injected from a syringe at a resolution as high as before. If the resolution is reduced, even a smaller amount of the drug injected can be evaluated.”
A skill/tip enabling elevation of the polarization rate of nuclear spin was made available many years ago. One such skill is dynamic nuclear polarization (DNP) developed in the 1950s.
This method utilizes the feature that in the case of a magnet with 660-fold stronger electron spin than the nuclear spin of hydrogen, the direction of the magnetic force is more likely to be aligned with the electron spin than with the nuclear spin if compared under an identical environment. In a static magnetic field, both electron spin and nuclear spin undergo motion as if a ‘top’ (a child’s toy) turns while shaking its neck (“precession”). If a microwave with the wavelength resonating with the electron spin is irradiated under such a condition, the direction of the electron spin will be adopted by the nuclear spin. With DNP, the nuclear spin polarization rate can be principally increased 660-fold.
This approach, however, involves disadvantages such as the necessity of cooling the sample to an ultralow temperature (minus 273C) to avoid the thermal influence and the need of an ultraconductive magnet to create a strong magnetic field. Quantum is delicate and our world is full of noise.
“Room temperature hyper-polarization” achieved for the first time
A technique called “triplet DNP” has been studied for many years by the research group led by Prof. Masahiro Kitagawa (Osaka University).
In some organic compounds, such as pentacene, two electrons usually having reverse spins to each other form a pair, resulting in off-setting of their magnetic forces. However, if a laser is applied to these compounds, their electron orbit becomes unstable, resulting in the manifestation of magnetic nature for a while. These electron spins have a high polarization rate due to the principle of quantum mechanics. Here arises an idea to increase the nuclear spin polarization rate of the sample to be investigated by adding a small amount of such a substance to the sample to induce DNP during a very short period of time (1/50,000 seconds) after laser irradiation. This technique does not depend on temperature and thus has an advantage that it is applicable at room temperature. Associate Professor Negoro was involved in this research as a teaching assistant, succeeding in 2014 in increasing the polarization rate to 34% (10,000-fold higher than the rate with conventional MRI). Associate Professor Negoro named this technique “room temperature hyper-polarization.” Now, research on room temperature hyper-polarization has expanded to other countries, including Germany, Switzerland and the USA.
Real-time evaluation of anti-cancer drug efficacy
When asked about concrete advantages of improving the sensitivity of MRI, Associate Professor Negoro answered: “Let’s take an example of injecting a substance called pyruvic acid into a blood vessel in a nuclear spin-aligned form. Pyruvic acid is converted into lactic acid in cancer cells and into alanine in healthy cells. If its course is followed by MRI, its state at varying occasions can be known on a real-time basis, e.g., ‘Now, lactic acid has increased’ or ‘xxx seconds were taken for the lactic acid to increase.’ In this way, we can check which drug is manifesting efficacy in a timely manner. This will shorten the time taken for assessment of responses to anti-cancer agents to only about 1 day, although it conventionally requires one month of check as to whether the cancer has grown in size using positron emission tomography, for example. If room temperature ultra-polarization for a randomly selected substance is enabled, the size and cost of the apparatus needed for such assessment may also be reduced. If other molecules are used as sensors, in addition to pyruvic acid, this technique is expected to be applicable to other diseases. Now, our research team, including physicians and other specialists, is continuing technical development of the technology which will enable delivery of drugs to the affected sites of human body while retaining their nature as quantum (nuclear spin direction aligned).”
Quantum sensing will alter the common sense about material analysis
Application to the field of chemistry is also promising.
“NMR is now utilized in extensive fields, including material analysis, protein structure and analysis. The current DNP NMR is designed to be used at ultra-low temperature levels. It enables the observation of substances only under the so-called extreme environments. In 2019, however, we increased the sensitivity of an ordinary NMR spectroscopic device at room temperature by 200-fold through combining it with a triplet DNP device. This improvement allows, first of all, observation of the substance’s status at room temperature. Furthermore, at this higher sensitivity, analysis of trace materials, which conventionally required accumulation of data through repeated measurements, can be completed by a one-shot action. Moreover, only minimal signals were caught at the interface between two different substances. If this interface can be analyzed, it will lead to the development of new functional materials such as rubber, films, catalysts and polyporous materials. Close attention is being paid also to the application of this technology to biological analyses such as membrane protein analysis.”
The cultivated quantum technology is sure to help us
Associate Professor Negoro was initially a researcher of quantum computers. Why did he begin to be engaged in research on MRI and NMR?
“There are many types of quantum computers. I was studying the methods for handling the state of nuclear spin (spin direction, etc.) as a unit of information (quantum bit). To this end, it is necessary to initialize the direction of nuclear spin, i.e., to align the direction of spins once. This resembles the process of initializing the status of Soroban (a Japanese version of an abacus). The beads constituting the Soroban are equivalent to nuclear spins. The magnetic force of nuclear spins is weak and is likely to disturbed by heat. I studied the triplet DNP technique to overcome this difficulty and achieved the polarization of 34%. To realize a quantum computer, a 99% polarization rate is needed. The path to this goal is still long. However, the polarization rate of 34% can also be viewed as a marked technological improvement and we cannot leave it unutilized. When I explored any way of utilizing this technology, I became aware of its application to new diagnosis with MRI and material analysis with NMR.”
He adds that he was affected also by the historical background.
In 1994, Shor, a US mathematician, demonstrated that a quantum computer will be able to achieve prime factorization (used for ciphers) in a very short period of time. Following this report, a quantum computer boom arose across the world. However, the initial enthusiasm subsided when a high hurdle against its realization was revealed. Before long, the “years of winter” prevailed in this research field. In contrast to the recent global trend of fierce competition in this field, the quantum computer field in the early half of the 2010s was treated like “research topics unknown as to when it will bear fruit.” It was difficult in those days to get funding or posts for this kind of research. Under such circumstances, many researchers were forced to shift their main focus to other topics that had “visible exits.” However, there was a fertile wide area beyond this half-deserted field.
The world has been changed by investment into dream stories
Slightly more than 100 years have elapsed since the birth of quantum mechanics, which pertains to the microworld such as molecules and atoms. The resulting technologies making use of the nature of quantum, such as semiconductors and lasers, have benefited mankind greatly. The quantum research being conducted actively at present, with the new year 2021 approaching, attempts to control and utilize the quantum state freely. This is termed “Quantum Technology 2.0.”
“Quantum computers are the starting point of quantum technology 2.0,” so says Associate Professor Negoro. He adds: “With companies, such as Google and IBM, having large investments into this field, we may say that quantum computers are now under the second boom. However, I am not sure as to whether we can realize this new computer under this boom alone. The winter may come again. In any event, numerous new technologies will be yielded from the challenging studies on large-scale quantum computing, just like the Apollo Program of sending humans to the moon which yielded new technologies. Just for this reason, I wish that the research into this field is not given up as a “dream story”, but is instead viewed as a promising field deserving long-term investment.”
He expressed his own determination to pursue a dream, saying “The research on the next-generation MRI will arrive at the current goal in 10 years. Then, my challenge will swing into full gear towards the goal of realizing a large-scale quantum computer.”
What is research for Associate Professor Negoro?
It changes the world and society. The world known to no one will become a world known to me alone. What is unknown to anyone may have applicability not yet identified. It is the privilege for the person having first discovered something to think “For what purposes will this be useful?” I wish to utilize this chance to change society.
■Specially Appointed Associate Professor (Full-time) Makoto Negoro
Institute for Open and Transdisciplinary Research Initiatives, Osaka University
- 2005: Graduation from Department of Electronic Physics, School of Engineering Science, Osaka University.
- 2007: Completion of the first semester of doctoral course, Osaka University Graduate School of Engineering Science.
- 2011: Completion of the second semester of the same course. Teaching Assistant specializing in System Creation at Osaka University Graduate School of Engineering Science.
- Since February 2019: Current position.
- 2020: Quantum and Life Science Group Leader at National Institutes for Quantum and Radiological Science and Technology (cross-appointment system). Ph.D. (Science).
(Interviewed in November 2020)