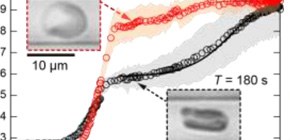
Resilient Red Blood Cells Need Fuel to Adapt their Shape to the Environment
International researchers led by Osaka University build a microfluidic system to measure the resilience of red blood cells after constriction
Red blood cells, also called erythrocytes, need a certain springiness to circulate through the body. Some capillary blood vessels are smaller than the diameter of an erythrocyte, which forces them to be folded as they squeeze through. Researchers have developed a new system for studying the resilience of red blood cells. This finding may lead to improved diagnosis and treatment of blood-related diseases, for example, septic shock and malaria.
To study how erythrocytes spring back into shape after deforming to pass through a constriction, an international team of researchers centered at Osaka University built what is called a “Catch-Load-Launch” microfluidic platform. The findings were recently published in Scientific Reports .
The experimental setup included a microchannel, in which a single erythrocyte could be held in place for any desired length of time before being launched into a wider section using a robotic pump, simulating the transition from a capillary into a larger vessel.
“The cell was precisely localized in the microchannel by the combination of pressure control and real-time visual feedback,” study coauthor Makoto Kaneko says. “This let us ‘catch’ an erythrocyte in front of the constriction, ‘load’ it inside for a desired time, and quickly ‘launch’ it from the constriction to monitor the shape recovery over time.”
Each erythrocyte has an internal cytoskeleton made of interlinking networks of filaments, including spectrin proteins, that contributes to its rigidity. Dynamic remodeling of this scaffold as the erythrocyte changes shape requires the cell to expend fuel, usually adenosine triphosphate (ATP). The researchers found that as the time the erythrocyte was held in the constricted region was increased—from 5 seconds all the way to 5 minutes—the time it took the cell to recover its normal shape also increased. For very short constriction times, the cells bounced back within 1/10 of a second. Conversely, it took approximately 10 seconds for cells to recover if they were held in the narrow segment longer than about 3 minutes.
The researchers next studied how the red blood cells’ recovery time was affected by a lack of ATP. Contrary to intuition, cells deprived of this fuel, ATP, took less time to recover.
Finally, the “Catch-Load-Launch” system was used to study the life-threatening condition known as septic shock. This can occur when bacteria invade the bloodstream and release endotoxins, which are known to affect cytoskeleton proteins. Patients in septic shock may suffer from reduced circulation inside the narrow blood vessels as the erythrocytes become too stiff. The same problem can be caused by Plasmodium falciparum, the parasite responsible for malaria.
The researchers exposed red blood cells to endotoxin from the bacteria Salmonella minnesota, and found the erythrocytes became stiffer and less resilient, like those starved of ATP.
“There is a great deal of evidence that relates certain diseases, including sepsis and malaria, to a decrease in the deformability of red blood cells,” lead author Hiroaki Ito says. “Such a stiffening can lead to a disturbance in microcirculation, and our ‘Catch-Load-Launch’ platform has the potential to be applied to the mechanical diagnosis of these diseased blood cells.”
Abstract
Large deformability of erythrocytes in microvasculature is a prerequisite to realize smooth circulation. We develop a novel tool for the three-step “Catch-Load-Launch” manipulation of a human erythrocyte based on an ultra-high speed position control by a microfluidic “robotic pump”. Quantification of the erythrocyte shape recovery as a function of loading time uncovered the critical time window for the transition between fast and slow recoveries. The comparison with erythrocytes under depletion of adenosine triphosphate revealed that the cytoskeletal remodeling over a whole cell occurs in 3 orders of magnitude longer timescale than the local dissociation-reassociation of a single spectrin node. Finally, we modeled septic conditions by incubating erythrocytes with endotoxin, and found that the exposure to endotoxin results in a significant delay in the characteristic transition time for cytoskeletal remodeling. The high speed manipulation of erythrocytes with a robotic pump technique allows for high throughput mechanical diagnosis of blood-related diseases.
Fig. 1 | Microchannel connected to a robotic pump. Feedback loop between high-speed vision and actuator enables real-time on-chip manipulation of a red blood cell (RBC). RBC deforms inside the narrow path for a specified time.
Fig. 2 | Drastic difference in red blood cell (RBC) deformability in response to different loading times. The shape recovery after 180 s deformation is drastically slower, indicating the internal remodeling of cytoskeletons.
To learn more about this research, please view the full research report entitled "Mechanical diagnosis of human erythrocytes by ultra-high speed manipulation unraveled critical time window for global cytoskeletal remodeling" at this page of the Scientific Reports website.
Related links