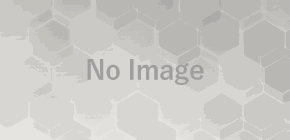
Clarification of mechanism of spontaneous recovery from paralysis after brain damage
Synopsis
Under the leadership of Professor YAMASHITA Toshihide and Assistant Professor UENO Masaki of the Graduate School of Medicine , Osaka University, an Osaka University team of researchers has succeeded in clarifying a mechanism of motor function recovery after brain damage in experiments using mice.
The impairment of neural networks by brain damage such as cerebral vascular disease or brain injury causes severe neurological symptoms such as limb paralysis. However, lost function may recover to some extent with time. It has been thought that undamaged neural networks compensate for lost functions, but the reality of how this was done was not known.
Through the use of mice, this group demonstrated that even after brain damage in major areas, the formation of new neural networks brings about a degree of spontaneous recovery of neural function and clarified mechanisms for such.
When the one side of the motor cortex*1 was damaged, the corticospinal tract*2 for motor function is impaired and severe motility disorder is caused in legs on the contralesional side, but this motility disorder recovers in small steps.
When this group looked at the the corticospinal tract on the undamaged side, they saw the tract had formed new neural networks in the spinal cord and thus recovered some motor function. (Figure 1)
Brain-derived neurotrophic factors (BDNF)*3 expressed in the spinal cord were found to promote the formation of these new circuits.
If it is found that a similar mechanism can function in primates such as humans and monkeys based on this group's discovery, such a finding will lead to the development of new treatment methods and the building of a scientific foundation for rehabilitation.
This research result was published on online news at the web site of British science magazine Brain on March 21, 2012 GMT.
This research was conducted as part of the research project, "Clarifying the mechanism of restoration of neural network and functional recovery after injuries to the central nervous system" (project leader: YAMASHITA Toshihide), in the research area headed by OZAWA Seiji, "Elucidation of the Principles of Formation and Function of the Brain Neural Network and Creation of Control Technologies", JST Strategic Basic Research Programs, Core Research for Evolutionary Science and Technology (CREST) of the Japan Science and Technology Agency (JST).
Background
Brain damage such as cerebral vascular disease or brain injury destroys neural networks, causing severe impairment to neurological functions such as recognition, movement, and sensation. However, the reality of such damage is that effective treatment methods have not been established. It was long believed that central nerves such as the brain and spinal cord could not be regenerated once they were damaged, therefore, spontaneous functional recovery after injuries to the central nervous system gained little publicity and not been clarified scientifically. In reality, however, some lost functions may recover to differing extents through rehabilitation and training.
The hypothesis had been proposed that undamaged nerve circuits may compensate for the lost functions, but how nerve circuits change and compensate for the function and in what way such mechanisms worked were not known. Clarification of the voluntary recovery mechanism of the brain will greatly contribute to the development of treatment methods for aftereffects following brain damage.
Findings
This group conducted experiments in which they damaged one side of the motor cortex of mice and destroyed one side of the cerebral cortex. In these experiment models, severe motility disorder was shown in the legs of the contralateral side of the damage.
Usually, the motor axons*4 of the corticospinal tract cross beneath the brain and extends to the spinal cord. (Figure 2: humans. The same is true for mice.) For this reason, if an injury happens on the left side of the brain, the right leg will be paralyzed. However, a few weeks after the injury, gradual and partial recovery of the motor function was observed. Then the group examined how this spontaneous recovery had happened.
In these brain injured models, the corticospinal tract extending from the motor cortex on the opposite side of the injury remained, the group paid attention to changes in the corticospinal tract. When they looked at this corticospinal tract using a neuronal anterograde tracer*5 to see the whole axon. Following brain injury, this corticospinal tract was found to have many branches at the vervical cord. These branches usually extend toward the same side of the spinal cord, but after brain injury, some of them extended to the opposite side beyond the center of the body. (Figure 3) The branches extended to the opposite side connected with spinal interneurons and connected with lower motor neurons. (Figure 2) In this way a neural network from the cerebral cortex to the muscles was established.
By exciting the motor cortex on the undamaged side with an electric stimulus, muscle movements in the forefoot on the paralyzed side were triggered. When this neural network was cut, the recovered motor function was lost again. (Figure 4)
These experiment results show that the cerebral cortex on the undamaged side compensate for the functions of the damaged side and the paralyzed forefoot became capable of movement. It demonstrated that the new neural circuits formed in the cervical cord contributed to spontaneous recovery of motor function.
Furthermore, this research group aimed to identify factors for causing the formation of such new circuits. As a result, the group found BDNF expression in the spinal interneurons and TrkB receptor expression on branches of the corticospinal tract. Then the group suppressed expression of BDNA or TrkB after brain injury, the formation of new circuits decreased and the recovery of motor function was reduced as well. In contrast, the additional expression of BDNF increased the formation of circuits. From this evidence, it was clarified that BDNF-TrkB signaling is needed for the formation of new circuits after brain injury. (Figure 5)
Future development
This discovery will lead to the clarification of a mechanism of recovery of neural symptoms caused by cerebral vascular disorder and brain injury in humans and monkeys possessing higher neural network. (Figure 6) It is known that, in primates, part of corticospinal tract goes down without crossing and it may contribute to effective functional recovery following brain damage. Progress in this research will clarify the mechanism of self-recovery of the brain. Furthermore, it will lead to the development of therapeutic methods for promoting the formation of compensatory neural networks and the building of a scientific foundation for methods and techniques of rehabilitation.
Figure 1
Figure 2
Figure 3
Figure 4
Figure 5
Figure 6
Notes
- Motor cortex -- The cerebral cortex is a layer of gray matter covering the surface of the brain. The cortex has neuron cells, controlling a variety of neuron functions. Functionally, the cerebral cortex is divided into many areas. The motor cortex area controls voluntary motions.
- Corticospinal tract -- Corticospinal tract is a major neural circuit for controlling voluntary motion. The tract extends from the cerebral cortex to the spinal cord. Many neurons are locally present in the motor cortex and extend fibers all the way down the spinal cord. As the corticospinal tract crosses over to the contralateral side in the lower bulbar of the spinal cord, neurons on the left side of the cerebral cortex control movements of limbs on the right side.
- BDNF -- One of the brain-derived neurotrophic factors; it has functions to promote the survival and growth of neurons. It functions by combining with TrkB receptor.
- Axon -- is a nerve fiber extending from the neuron, conducting electrical impulses away from the neuron's cell body.
- Neuronal anterograde tracer -- is a compound that, when injected in a neuron, reaches the terminal of the axon thereby revealing a picture of the connection of the axon. This enables researchers to observe neural networking directly.
- Spinal interneurons -- Neurons in the spinal cord that carry signals transmitted in the neural network responsible for motor function and sensation.
Related information
- Thesis : " Intraspinal rewiring of the corticospinal tract requires target-derived brain-derived neurotrophic factor and compensates lost function after brain injury " (Corticospinal tract reorganization in the spinal cord through BDNF, contributing to functional recovery following brain damage) published in online news at the website of British science magazine Brain on March 21, 2012 GMT.
Related link: