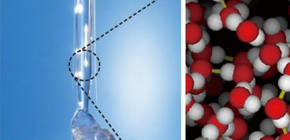
Solution to Mysterious Behavior of Supercooled Water
Osaka University-led researchers characterize the unexplained diffusion and viscosity behavior of supercooled water with new, highly accurate computer simulations
When Einstein was working toward his PhD, he was among the first to explain how particles exhibit random motions in fluids. Diffusion is an important physical process and the Stokes–Einstein relationship describes how particles diffuse through a fluid based on the hydrodynamic theory. But mysteriously at low temperatures below the melting temperature, something changes in supercooled liquids and the resulting highly viscous glassy behavior can no longer be explained by the simple Stokes–Einstein relationship.
Now, two researchers from Osaka University and Nagoya University have simulated supercooled water in unprecedented detail to explain the anomalous behavior at low temperatures. They recently published their findings in Science Advances.
“Most liquids obey the Stokes–Einstein equation over a wide range of temperatures, but some unexpected changes in behavior are found in supercooled water and other glassy materials,” coauthor Kang Kim, of Osaka University, says. “Breakdown of Stokes–Einstein behavior suggests some kind of anomalous molecular motions even in a liquid state, but it’s not clear what those behaviors are.”
The simple Stokes–Einstein relationship is based on arguments about how molecules move randomly at the microscopic level. But in supercooled water, molecules start to slow down irregularly. The researchers showed through simulations that some regions of the water become affected more than others, forming hydrogen bonds heterogeneously with partial solidification.
Water molecules move through the viscous supercooled water in jumps related to hydrogen bond breaking. The erratic timing of this kind of movement is not accounted for by the Stokes–Einstein equation.
Their simulations allowed them to examine how the supercooled water hydrogen bonding network changed over time. Their modelling clearly showed that an intermittent hydrogen bond breaking timings contributed to the breakdown of Stokes–Einstein behavior.
“There are interesting physical implications of these findings as the Stokes–Einstein violation is regarded as a hydrodynamic anomaly of many glassy materials systems,” Kim says. “Our simulations help to answer questions about what happens in pure supercooled water and could also help to explain other dynamic behaviors in other technologically important glassy materials.”
Figure 1 . (Left) Supercooled water is frozen with shocks (Copyright: Takeshi Fujino, KOWA Corporation, Niigata, Japan) (Right) Snapshot of supercooled water calculated from our computer simulations (red: oxygen, white: hydrogen, yellow bond: hydrogen-bond). (credit: Osaka University)
Figure 2. In the supercooled water, two hydrogen-bonded water molecules (red: oxygen, white: hydrogen) exhibit jumping motions with hydrogen-bond breakage. The other water molecules are colored by light blue. The hydrogen-bonds are colored by yellow. (credit: Osaka University)
To learn more about this research, please view the full research report entitled “ Identifying time scales for violation/preservation of Stokes-Einstein relation in supercooled water ” at this page of the Science Advances website. (DOI: 10.1126/sciadv.1700399)
Related link