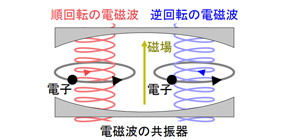
Quantum shift shows itself in coupled light and matter
Rice University scientists corral, quantify subtle movement in condensed matter system
A team led by Rice University scientists used a unique combination of techniques to observe, for the first time, a condensed matter phenomenon about which others have only speculated. The research could aid in the development of quantum computers.
The researchers, led by Rice physicist Junichiro Kono and graduate student Xinwei Li, observed and measured what's known as a Bloch-Siegert shift in strongly coupled light and matter.
Results of the complicated combination of modeling and experimentation are the subject of a paper in Nature Photonics . The technique could lead to a greater understanding of theoretical predictions in quantum phase transitions because the experimental parameters used in the Rice experiments are highly adjustable, according to Kono. Ultimately, he said, it may help in the development of robust quantum bits for advanced computing.
The Bloch-Siegert shift, a theory born in the 1940s, is a quantum interaction in which counter-rotating fields are able to interact. But such interactions have been difficult to detect.
The theory suggested to Kono and Li that it might be possible to detect such a shift when a light field rotating in one direction strongly couples with a matter-bound electron field rotating in the opposite direction. These interactions have proven difficult to create without the unique tools assembled by the Rice-led team.
"Light and matter should not resonate with each other when they are rotating in opposite directions," Kono said. "However, in our case, we proved they can still strongly couple, or interact, even though they are not resonating with each other."
Kono and his colleagues created the resonance frequency shift in a two-level electron system induced by coupling with an electromagnetic field inside a cavity even when the electrons and field are rotating in opposite directions – a truly surprising effect that occurs only in a regime where light and matter are mixed together to an extreme degree.
In this case, the levels are those of two-dimensional electrons in solid gallium arsenide in a strong perpendicular magnetic field. They hybridize with the "vacuum" electromagnetic field in the cavity to form quasiparticles known as polaritons . This vacuum-matter hybridization had been expected to lead to a finite frequency shift, a vacuum Bloch-Siegert shift, in optical spectra for circularly polarized light counter-rotating with the electrons. The Rice team can now measure it.
"In condensed matter physics, we often look for new ground states (lowest-energy states). For that purpose, light-matter coupling is usually considered an enemy because light drives matter to an excited (higher-energy) state," Kono said. "Here we have a unique system that is predicted to go into a new ground state because of strong light-matter coupling. Our technique will help us know when the strength of light-matter coupling exceeds a certain threshold."
The research builds upon a strong vacuum field-matter coupling in a high-quality-factor cavity the lab first created and reported in 2016 . The results at the time only hinted at the presence of a Bloch-Siegert shift. "Experimentally, we just demonstrated the new regime," Li said. "But here, we have a very deep understanding of the physics involved."
Kono and Li credited physicist Motoaki Bamba of Osaka University for providing a theoretical basis for the discovery and Katsumasa Yoshioka of Yokohama National University and a former visiting scholar at Rice for providing a device to produce circularly polarized light in the terahertz range of the electromagnetic spectrum.
The lab used the light to probe the shift in an ultra-high quality, two-dimensional electron gas supplied by Purdue University physicist Michael Manfra and set in a gallium arsenide quantum well (to contain the particles) under the influence of a strong magnetic field and low temperature. A terahertz spectroscope measured activity in the system.
"Linearly polarized light means an alternating current electric field that is always oscillating in one direction," Kono said. "In circularly polarized light, the electric field is rotating." That allowed the researchers to distinguish between left- and right-rotating electrons in their vacuum-bound condensed matter in a magnetic field, and from that, measure the shift.
"In this work, both theoretically and experimentally, we demonstrated that even though the electron is rotating this way and the light is rotating (the other) way, they still strongly interact with each other, which leads to a finite frequency shift known as the Bloch-Siegert shift," Kono said.
Observing the shift is a direct indication that ultra-strong light-matter coupling invalidated the rotating wave approximation , he said. "That approximation is behind almost all light-matter interaction phenomenon, including lasers, nuclear magnetic resonance and quantum computing," Kono said. "In any resonant light-matter interaction, people are satisfied with this approximation, because the coupling is usually weak. But if the coupling between light and matter is strong, it doesn't work. That's clear evidence that we are in the ultra-strong coupling regime."
Co-authors of the paper are Rice postdoctoral researcher Weilu Gao and graduate student Minhan Lou of Rice, Rice alumnus Qi Zhang of Argonne National Laboratory and graduate student Saeed Fallahi and visiting scholar Geoff Gardner of Purdue. Kono is a professor of electrical and computer engineering, of physics and astronomy, and of materials science and nanoengineering. Manfra is the Bill and Dee O’Brian Chair Professor of Physics and Astronomy at Purdue. Bamba is an associate professor at Osaka. Yoshioka is a teaching assistant at Yokohama.
The National Science Foundation, the Army Research Office, the Department of Energy Office of Basic Energy Sciences, the PRESTO program of the Japan Science and Technology Agency and the ImPACT program of the Government of Japan's Council for Science, Technology and Innovation supported the research.
Read the open-access paper at http://dx.doi.org/10.1038/s41566-018-0153-0
Kono Laboratory: http://kono.rice.edu
Motoaki Bamba: http://empty.matrix.jp
Manfra Group: http://manfragroup.org
Katsumasa Yoshioka: http://katsu-yoshioka.main.jp
Rice Department of Electrical and Computer Engineering: https://eceweb.rice.edu
Rice Department of Physics and Astronomy: http://www.physics.rice.edu
Rice Department of Materials Science and NanoEngineering: https://msne.rice.edu
Video:
CAPTION: An electron (blue sphere) travels in a circular orbit in a DC magnetic field (B_dc). When an incoming light wave (E_ac) interacts with it, there is a component in the light wave whose electric field rotates in the same direction with the electron motion (red arrow on the left-hand side) and thus resonantly interacts with it – that is, the electron rapidly gains energy. The other component of the electric field rotates in the opposite direction with the electron (red arrow on the right-hand side), whose effect is typically negligible. However, when the electron and light wave mix to an extreme degree, the interaction effect can manifest as a Bloch-Siegert shift. (Credit: Xinwei Li/Kono Lab at Rice University)
Researchers at Rice University, including graduate student Xinwei Li, have observed and measured a Bloch-Siegert shift in strongly coupled light and matter in a vacuum. The project could aid in the development of quantum computers. (Credit: Jeff Fitlow/Rice University)
Xinwei Li, left, and Junichiro Kono. (Credit: Jeff Fitlow/Rice University)
A simplified schematic shows the basic idea behind a Rice University experiment to detect a Bloch-Siegert shift in strongly coupled light and matter. In this illustration, a light field rotating in the opposite direction to an orbiting electron still interacts with the electron in a cavity, in this case the empty space between two mirrors. The influence of resonance on the counter-rotating element defines the shift. (Credit: Xinwei Li/Kono Lab at Rice University)